Quark Gluon Soup
Physicist Rosi Reed investigates elements that hold the universe together
For a moment after the Big Bang, just a few millionths of a second, the universe was filled with an incredibly hot, dense liquid composed of particles moving at near-light speed. This mixture was dominated by quarks—fundamental bits of matter—and by gluons, carriers of the force that normally “glues” quarks together into familiar protons and neutrons and other particles. A quark-gluon plasma (QGP) occurs when the temperature is so high — an average of 4 trillion degrees Celsius — that individual protons and neutrons comprising atoms melt, and this event is the focus of research by high energy physicist Rosi Reed.
Funded by the National Science Foundation’s Major Research Instrumentation program, Reed’s research explores hardware development and data analysis. Working at the Relativistic Heavy Ion Collider (RHIC), which is located at Brookhaven National Labs (BNL) on Long Island, New York, she has been involved in the development of two different detectors—the Event Plane Detector (EPD) for STAR and the sPHENIX Event Plane Detector (sEPD).
“With nuclear force, we can't pull things apart without a giant collider,” says Reed, associate professor of physics. “The range of the force is on the order of 10 to minus 15 meters. You can't test it at a great distance either. So, it requires a lot of energy to break up the various nucleons into their constituent parts, and it requires very fast timing to measure what's happened. It's one of the more complicated forces to study outside of gravity essentially.”
She uses particle jets as probes to analyze QGP to answer fundamental questions about the nature of quark-gluon interactions. Particle jets are formed when a high momentum quark or gluon splits into a column of particles, which create subatomic particles called hadrons and are measured by the detectors. When this particle travels through the QGP, it will lose energy to the medium in a process called jet quenching. The modification of the jets due to the medium depends on the details of the medium such as its temperature, the size of the fluctuations within it, and the geometry of the QGP droplet. Comparing models and data from proton-proton collisions, the properties of the quark-gluon interaction can be understood.
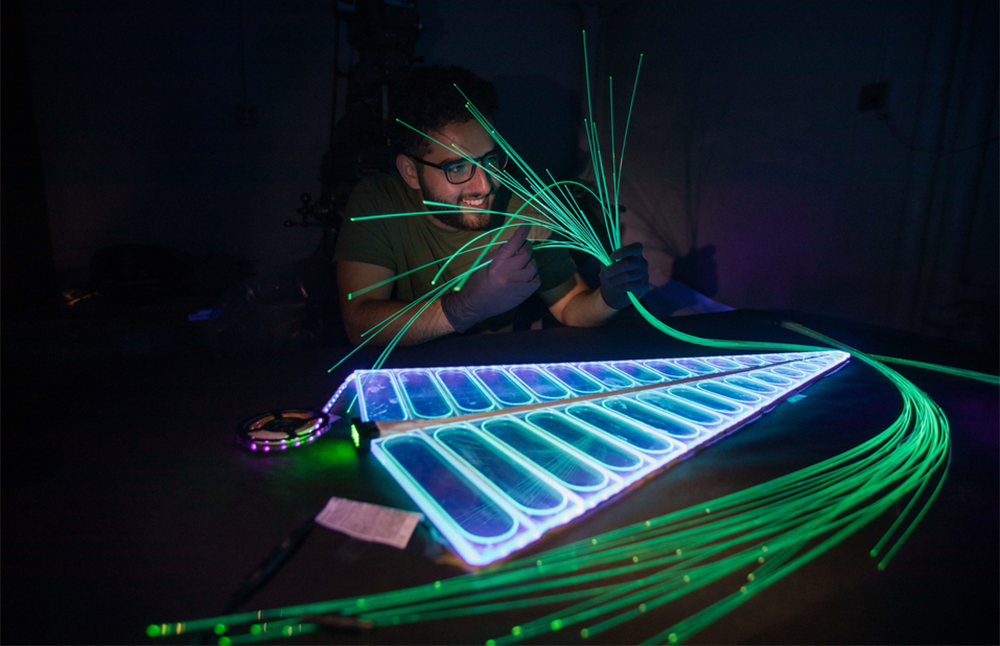
Previously, Reed installed a detector in the STAR experiment to measure charge particle distributions. This past spring, a detector was installed at sPHENIX, which is a massive cylindrical detector wrapped around a beam. Reed’s detector was attached along either end, and it is segmented so that scientists can examine the charged particle distributions. When a collision happens in the detector, Reed and her colleagues want to know the details of how the ions collided.
“They don't have to collide head-on,” she says. “They can collide at an angle, and we need to know did they just barely touch each other? Did they hit each other really? If they hit each other head-on, you get more particles out. If they barely touch each other, you get a few. But what we also need to know is the angle of this collision. For my project, we've made it possible to measure certain quantities with a high precision that weren't going to be possible without my detector.
When scientists smash together these ions, a tiny drop of matter forms so hot that the protons and neutrons melt. It's like quark gluon soup, Reed says. And one of the questions is how do quarks and gluons interact with that soup? One of the main questions of sPHENIX is how a quark or gluon interacts with this quark gluon soup. Reed’s detector allows scientists to understand precisely how hard these ions have struck each other, whether they hit each other head-on or peripherally and what the angle is.
“In the collision, you might have something that's moving through the rest of the particles with a higher momentum and it loses energy as it moves through,” Reed says. “And so that's one of the main questions of sPHENIX, how does a quark or gluon interact with this quark gluon soup? My detector allows us to understand the precision of how hard these ions have hit each other, so whether they hit each other head on or peripherally and what the angle is, we know exactly the orientation of this drop. When we know this particle went through the long way or the short way, we can compare those results with a great deal of precision. And then you can understand this by comparing it to models of strong nuclear force. It essentially allows a whole suite of measurements that weren't possible without it.”