Modeling Electronic Processes
Lisa Fredin employs quantum mechanics to examine the chemical physics of catalytic materials.
With a changing climate, there is a need to develop more sustainable, environmentally friendly materials. Focusing on the development of models at the edge of theory and experiment, quantum chemist Lisa Fredin explores the chemical physics of catalytic materials to improve our fundamental understanding of emerging substances.
Fredin, assistant professor of chemistry, uses quantum mechanics to study the photochemical, electronic and magnetic properties of materials and improve scientists’ understanding of the processes involved during catalysis, charge and energy transfer, and electron or hole transport in complex electronic systems. Her team uses supercomputing power and method development to increase the reliability of experimental comparison and theoretically predicted materials from atomic to nanoscale. From this, they develop models to investigate fundamental electronic processes.
Supported by the National Science Foundation (NSF), she and her team use theoretical quantum mechanics to understand the properties of chemical systems. Part of the group’s work seeks to understand the characteristics of new chemical structures, such as new molecular systems pr nanoparticles. The other part is theoretical development using quantum mechanics.
“Quantum mechanics is a theory in science that has been proven over and over to be exactly true,” says Fredin, who also holds a Sloan Fellowship. “The problem is we solve the equations of quantum mechanics using a computer. And when we do that, we have introduced assumptions into the system. We cannot solve the equation of quantum mechanics exactly. So, the theory is exact, but the way we solve it is not. When we do that, we have introduced error into our understanding. And we have to, therefore, solve quantum mechanics multiple ways to make sure that the error we've introduced hasn't changed the chemistry answer. That leads to theoretical developments, how to build better models, how to use computers more efficiently.
My group calculates some of the largest structures that people can even run on supercomputers at this point in time. That means we have to make sure that we are both numerically accurate, but also chemically accurate.”
One of the most common industrial uses involving chemistry is developing catalysts. “We try to change certain chemicals into other chemicals. We try to take polymer waste or plastic waste and turn it into pharmaceuticals or small molecules that are useful for things,” she adds.” We try to create new polymers that can degrade out in the sunlight so that we don't fill up landfills. Understanding how to speed up or control reactions is the chemistry of catalysis. And a lot of that industrially happens on nano-sized systems because you get a lot of surface area for the amount of material you have. So, it's very cost-effective.”
"My group calculates some of the largest structures that people can even run on supercomputers at this point in time. That means we have to make sure that we are both numerically accurate, but also chemically accurate."
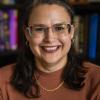
Enter Fredin’s laboratory and you can forget the stereotypical chemistry environment. Fredin’s lab is a room of computers, connected to the high-performance computing center in Lehigh’s Fairchild-Martindale Library. “This is one of the best things about Lehigh, why I came here. It's because the provost and the dean support the staff that run that facility,” she says.
The group’s work allows Fredin the opportunity to partner with experimentalists working on both inorganic and organic materials, bridging physical chemistry, material science and nanotechnology. Her most recent work involves teaming with colleagues at Lehigh and other institutions as part of a $26 million NSF grant to develop solutions to deal with the reclamation and repurposing of high-global warming potential (GWP) legacy refrigerants. These solutions are needed to prevent millions of metric tons of high-GWP refrigerants from leaking and illegally venting into the atmosphere.
Fredin is part of the team that is designing new light mediated tracking of refrigerants so that pollution can be tied back to particular companies, as well as designing new catalysts that efficiently repurpose legacy refrigerants into commodity chemicals that are in high demand in medicine and polymer industries. Her calculations provide insights into why the current catalysts work and how to design more selective light driven reactions.
Working at the boundaries of various types of chemistry, the Fredin group seeks to uncover insights into the fundamental properties of a broad range of atomic to nanoscale materials and build computational models that are both descriptive and predictive.
“We're looking at a computer and asking the computer a scientific question, and you have to ask the question right, and you have to set it up correctly. All of those are the same as running an experiment. You have to set up the experiment, you have to control all the variables. We have the same thing, it's just that in the end of the day, the final experiment is run by the computer.”